From Start To Finish: Inside The Lithium Battery Manufacturing Process
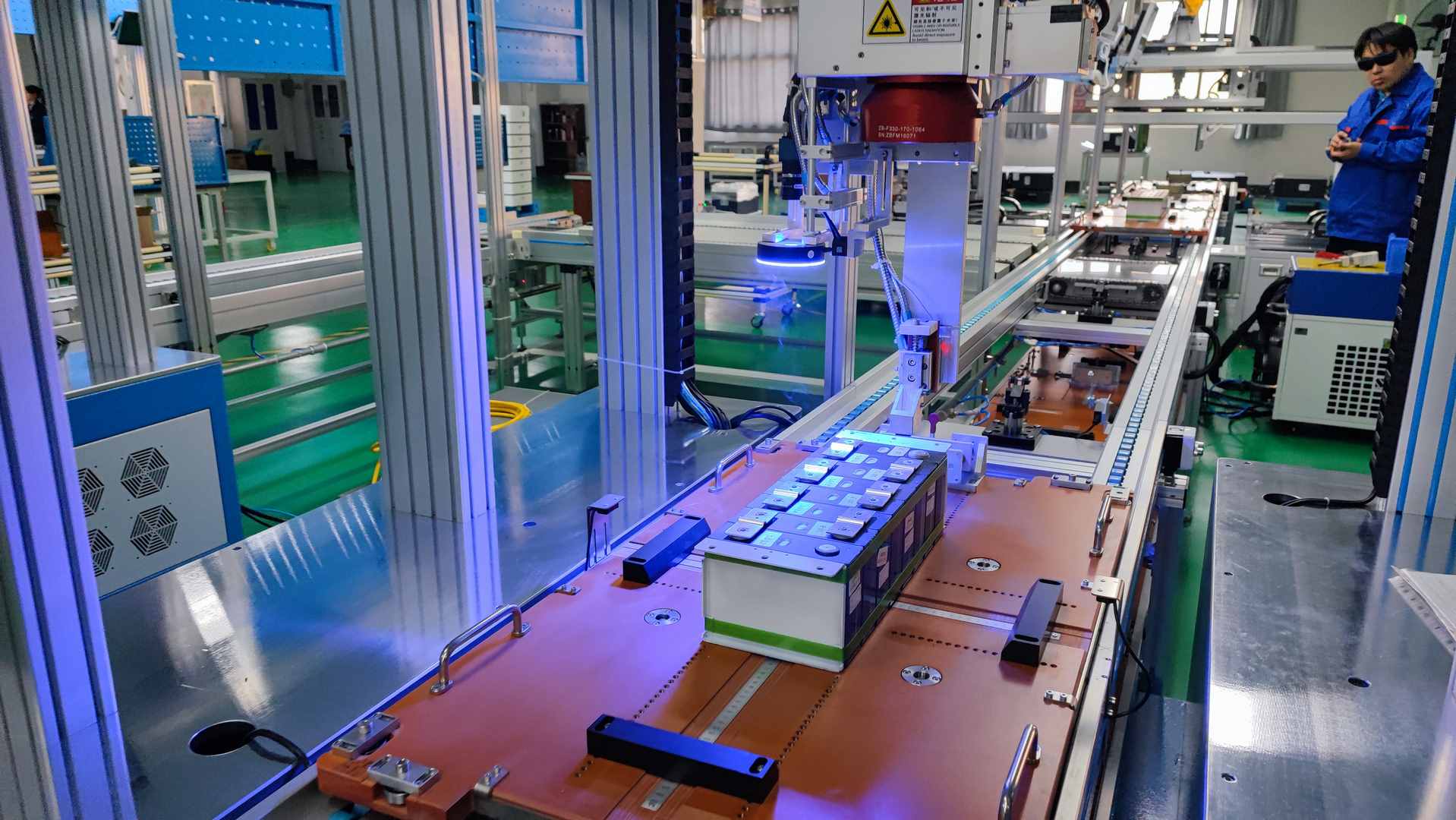
Lithium batteries are widely used in various applications, including portable electronics, electric vehicles, and renewable energy storage systems. To meet the increasing demand for these batteries, efficient manufacturing processes have been developed to ensure high-quality and cost-effective production.
Have you ever wondered how lithium batteries, the power source for our smartphones, electric vehicles, and even some renewable energy systems, are manufactured from start to finish?
In this article, we will take you on a journey through the complex and intricate process of lithium battery manufacturing, highlighting each key stage involved in creating these powerful and portable energy storage devices.
Section1: Raw Material Extraction and Preparation
The raw material acquisition involves sourcing and obtaining the necessary materials for battery production, such as lithium, cobalt, and nickel. Lithium salts, the key ingredient, are typically extracted from brine deposits or hard rock minerals through a combination of mining and chemical processes.
On the other hand, other battery materials like cathode, anode, and electrolyte are sourced from reliable suppliers, ensuring their quality and consistency to meet the demanding requirements of battery production.
(1) Extraction of Lithium-Rich Ores
The first step in the lithium battery manufacturing process involves extracting lithium-rich ores from mining sites. This is a crucial step as it provides the raw material necessary for the production of lithium batteries.
Here are three key aspects related to the extraction of lithium-rich ores:
- Mining Techniques: There are several mining techniques employed in the extraction of lithium-rich ores. One common method is open-pit mining, where large quantities of soil and rock are excavated to reach the ore deposits. Another technique is underground mining, which involves tunneling into the earth to access deeper ore bodies. Each method has its own advantages and challenges, depending on factors such as geological conditions and environmental considerations.
- Ore Processing: Once the lithium-rich ores are extracted, they undergo further processing to extract valuable minerals like lithium carbonate or lithium hydroxide. The processing methods can vary based on factors such as ore grade and desired end products. Common techniques include crushing and grinding the ore into finer particles, followed by chemical treatments like flotation or leaching to separate and concentrate the desired minerals.
- Environmental Impacts: The extraction of lithium-rich ores can have significant environmental impacts if not properly managed. Mining activities can result in land degradation, water pollution, and greenhouse gas emissions. To mitigate these impacts, various measures need to be implemented, such as reclamation plans for mined areas, water treatment systems to minimize pollution, and the adoption of sustainable practices throughout the entire process.
(2) Refining and Purification of Lithium Carbonate
Refining and purification of lithium carbonate involves implementing various techniques to ensure the removal of impurities and the production of high-quality lithium carbonate. This step is crucial in the lithium battery production process as it affects the overall quality, cost, efficiency, and compliance with manufacturing regulations.
The first step typically involves dissolving the crude lithium carbonate in water to create a solution. This solution is then subjected to filtration or centrifugation to remove insoluble impurities such as solid particles.
Next, various chemical treatments are utilized to eliminate other impurities present in the solution. These treatments include precipitation reactions using reagents that selectively react with specific contaminants, resulting in their removal from the solution.
Once these impurities have been eliminated, the remaining liquid undergoes further treatment steps like evaporation or crystallization to concentrate and isolate pure lithium carbonate.
Quality control plays a vital role throughout this refining and purification process. Strict adherence to manufacturing regulations ensures that all impurity levels are within acceptable limits for battery applications.
Additionally, continuous monitoring through analytical techniques such as spectroscopy or chromatography enables manufacturers to identify any potential deviations from desired purity standards early on during production.
(3) Preparation of Cathode Materials
The preparation of cathode materials involves a meticulous series of steps to ensure the production of high-performance components that contribute to the advancement and success of lithium battery technology.
The first step in this process is selecting and preparing the active material, typically a metal oxide such as lithium cobalt oxide (LiCoO2), lithium manganese oxide (LiMn2O4), or lithium iron phosphate (LiFePO4).
The active material is then mixed with conductive additives such as carbon black or graphite to enhance electron transfer within the cathode.
This mixture is carefully blended to achieve a homogenous distribution, ensuring uniform performance across the electrode.
Once the active material and conductive additives are combined, they are coated onto a current collector, usually made of aluminum foil.
This coating process requires precision to achieve optimal thickness and uniformity.
After coating, the cathode undergoes a drying process to remove any solvents used during mixing and coating. This ensures that the final product has excellent stability and electrical conductivity.
(4) Production of Anode Materials
An essential step in the manufacturing of lithium-ion batteries involves the production of anode materials. The anode is one of the two electrodes in a battery and plays a crucial role in the overall performance and efficiency of the battery.
Typically, graphite is used as the primary material for anodes due to its excellent conductivity and stability.
The production process starts with selecting high-quality graphite powder, which is then mixed with binders and solvents to form a slurry. This slurry is then coated onto a copper foil substrate using a technique called doctor-blade coating or roll-to-roll coating. After coating, it undergoes drying and calendaring processes to ensure uniformity and improve adhesion.
Once the graphite electrode has been prepared, it goes through several additional steps to enhance its electrochemical properties.
One such step involves applying a thin layer of protective material, such as carbon nanotubes or silicon oxide, on top of the graphite surface. This protective layer helps prevent degradation during charging and discharging cycles by reducing side reactions between lithium ions and electrolytes.
Additionally, various additives can be incorporated into the anode material to further enhance its performance, such as conductive agents like carbon black or metal nanoparticles.
Overall, producing high-quality anode materials requires precise control over various parameters, including particle size distribution, binder content, coating thickness, and composition of additives. These factors directly impact the capacity, cycling stability, rate capability, and safety of lithium-ion batteries.
(5) Separator Manufacturing
Separator manufacturing is a critical stage in the production of lithium-ion batteries, as it involves the fabrication of a thin and porous material that acts as a physical barrier between the anode and cathode, allowing for the selective movement of lithium ions while preventing short circuits and enhancing battery safety.
The process begins with the selection of suitable raw materials such as polyethylene or polypropylene, which are commonly used due to their high melting points and chemical stability. These materials are then melted down and extruded into a film form using specialized equipment.
To ensure optimal performance, the separator film undergoes several additional treatments. First, it is subjected to stretching in both machine and transverse directions to enhance its mechanical strength and porosity. This stretching process aligns the polymer chains within the film, creating microscopic channels that facilitate ion transport while maintaining structural integrity.
Next, the separator is coated with a ceramic layer to further improve its thermal stability and prevent internal short circuits caused by contact with metallic particles from electrodes.
By following these precise manufacturing steps, manufacturers can produce separators that meet stringent quality standards while ensuring efficient ion transport within lithium-ion batteries.
Section 2: Electrode Preparation and Coating
This discussion focuses on the electrode preparation and coating process in the manufacturing of lithium batteries. It encompasses various key points such as mixing and blending active materials, slurry preparation and coating techniques, drying and calendaring processes, and quality control measures.
(1) Mixing and Blending Active Materials
During the mixing and blending stage of the lithium battery manufacturing process, various active materials are carefully combined in a controlled environment to create a homogeneous mixture.
This step is crucial as it ensures that the active materials, such as lithium cobalt oxide for the cathode and graphite or silicon for the anode, are evenly distributed throughout the battery. Achieving a uniform distribution is essential for optimal performance and efficiency of the battery.
To accomplish this, manufacturers employ specialized equipment like mixers or blenders that can handle large volumes of materials. These machines provide precise control over factors such as rotation speed, mixing time, and temperature to achieve consistent results. The active materials are typically mixed with binders and solvents to form a slurry-like consistency that can be easily applied onto current collectors during electrode coating. Maintaining a controlled environment free from contaminants is crucial during this stage to prevent any impurities from affecting the quality of the battery.
Here is an engaging table showcasing three key active materials used in lithium-ion batteries:
Active Material | Role in Battery | Advantages |
---|---|---|
Lithium Cobalt Oxide (LiCoO2) | Cathode material | High energy density, good cycling stability |
Graphite | Anode material | Excellent conductivity, low cost |
Silicon | Anode material | High capacity, the potential for higher energy density |
This table highlights how each active material contributes unique characteristics to improve battery performance. By understanding these components better, individuals may gain insight into how advancements in lithium battery technology can lead us toward a future where we enjoy greater freedom through portable electronic devices with longer-lasting power sources.
(2) Slurry Preparation and Coating Techniques
Cell sorting and grading is a critical step in the lithium battery manufacturing process, ensuring that only cells meeting the required specifications are used for further production.
Slurry preparation involves creating a mixture of active materials, binders, solvents, and additives to form a homogenous paste-like substance known as slurry. This slurry acts as a crucial element in the production of electrodes for lithium batteries.
To provide a comprehensive understanding of slurry preparation and coating techniques, let us delve into four key aspects:
- Slurry formulation: The first step in slurry preparation is carefully selecting the appropriate active materials based on their electrochemical properties and desired performance characteristics. Binders are then added to enhance cohesion within the electrode structure. The choice of solvents depends on factors such as viscosity control and drying characteristics. Lastly, additives are incorporated to improve adhesion between layers or enhance battery performance.
- Mixing methods: Achieving uniform dispersion of active materials within the slurry is paramount for consistent battery performance. Traditional methods involve mechanical stirring or ball milling to ensure thorough blending without damaging the particles' integrity. More advanced techniques like ultrasonication or high-shear mixing have also been introduced to achieve better dispersion of nanomaterials.
- Slurry coating: Once properly mixed, the slurry is applied onto current collector substrates through various coating techniques such as doctor blade coating or slot die extrusion methods. These processes allow for controlled deposition thicknesses while ensuring an even distribution across large surface areas.
- Drying and solidification: After application, solvent evaporation must occur efficiently without causing cracks or defects in the electrode film structure. Controlled drying conditions using ovens or continuous roll-to-roll systems enable the gradual removal of solvents while maintaining structural integrity.
(3) Drying and Calendaring Process
The drying and calendaring process is essential for achieving high-quality electrodes with optimal structural integrity and performance in lithium batteries. By carefully controlling these steps, manufacturers can produce electrodes that exhibit uniformity and consistency throughout their structure.
After the slurry coating step, the solvent present in the electrode needs to be removed through a drying process. This is typically achieved by subjecting the coated electrodes to high temperatures, causing the solvent to evaporate. Care must be taken during this stage to ensure uniform drying throughout the electrode, as uneven drying can lead to defects such as cracks or delamination.
Once dried, the electrodes undergo calendaring, which involves compressing them under high pressure to improve their mechanical properties. This step helps increase the density and thickness of the electrode while also improving its electrical conductivity.
Calendaring also helps eliminate any remaining solvents that may not have completely evaporated during drying. The pressure applied during calendaring ensures good contact between active materials and current collectors, optimizing electron transfer within the battery system.
Section 3: Cell Assembly and Formation
During the assembly line process for lithium batteries, various components are carefully integrated to create a functional cell. The cell assembly line is designed to streamline the production process and ensure consistency in the final product. It typically consists of multiple stations where different tasks are performed sequentially.
(1) Electrode Stacking and Winding
Electrode stacking and winding is a critical step in the assembly line process for lithium batteries, this process is essential for optimizing the performance and efficiency of lithium batteries.
The electrode stacking and winding process typically involves multiple layers of alternating positive and negative electrodes, separated by thin layers of separator material. The electrodes are made up of active materials, such as lithium cobalt oxide or graphite, that store and release electrical energy during battery operation.
Table: Electrode Stacking Techniques
Technique | Description |
---|---|
Jelly Roll | Continuous winding of electrodes and separator material around a central core to create a spiral structure |
Folding/Pleating | Folding or pleating of electrodes to increase their surface area before stacking them together |
Zigzag | Alternating zigzag arrangement of positive and negative electrodes to enhance overall battery capacity |
Cross-Stacking | Crosswise arrangement of multiple electrode stacks to increase energy density |
Co-Extrusion | Simultaneous extrusion of different electrode materials in one continuous strip |
(2) Separator Placement
Separator placement is a crucial step in the assembly line process for lithium batteries, as it involves positioning the separator material between the positive and negative electrodes to enable efficient ion transport during battery operation.
The separator plays a vital role in preventing direct contact between the electrodes, which could result in short circuits or other safety hazards. It acts as a physical barrier that allows only the flow of ions while blocking the movement of electrons. This ensures that the battery functions properly and efficiently.
Here are four important aspects related to separator placement:
- Material selection: The choice of separator material is critical as it determines its performance characteristics. Typically, separators are made from polymer materials such as polyethylene (PE) or polypropylene (PP). These materials offer high thermal stability, good mechanical strength, and excellent electrolyte wettability.
- Thickness control: The thickness of the separator plays a significant role in determining its ionic conductivity and overall battery performance. Precise control over separator thickness is essential to achieve optimal ion transport and prevent excessive resistance within the battery.
- Uniformity and alignment: During separator placement, ensuring uniformity and proper alignment is crucial for consistent battery performance across all cells. Any variations or misalignment can lead to localized hotspots or uneven ion distribution within the battery, affecting its overall efficiency.
- Adhesive properties: To hold everything together securely, an adhesive layer may be applied during separator placement. This adhesive should have good adhesion to both electrodes while maintaining flexibility to accommodate volume changes during charging-discharging cycles without compromising cell integrity.
(3) Introduction of Electrolyte
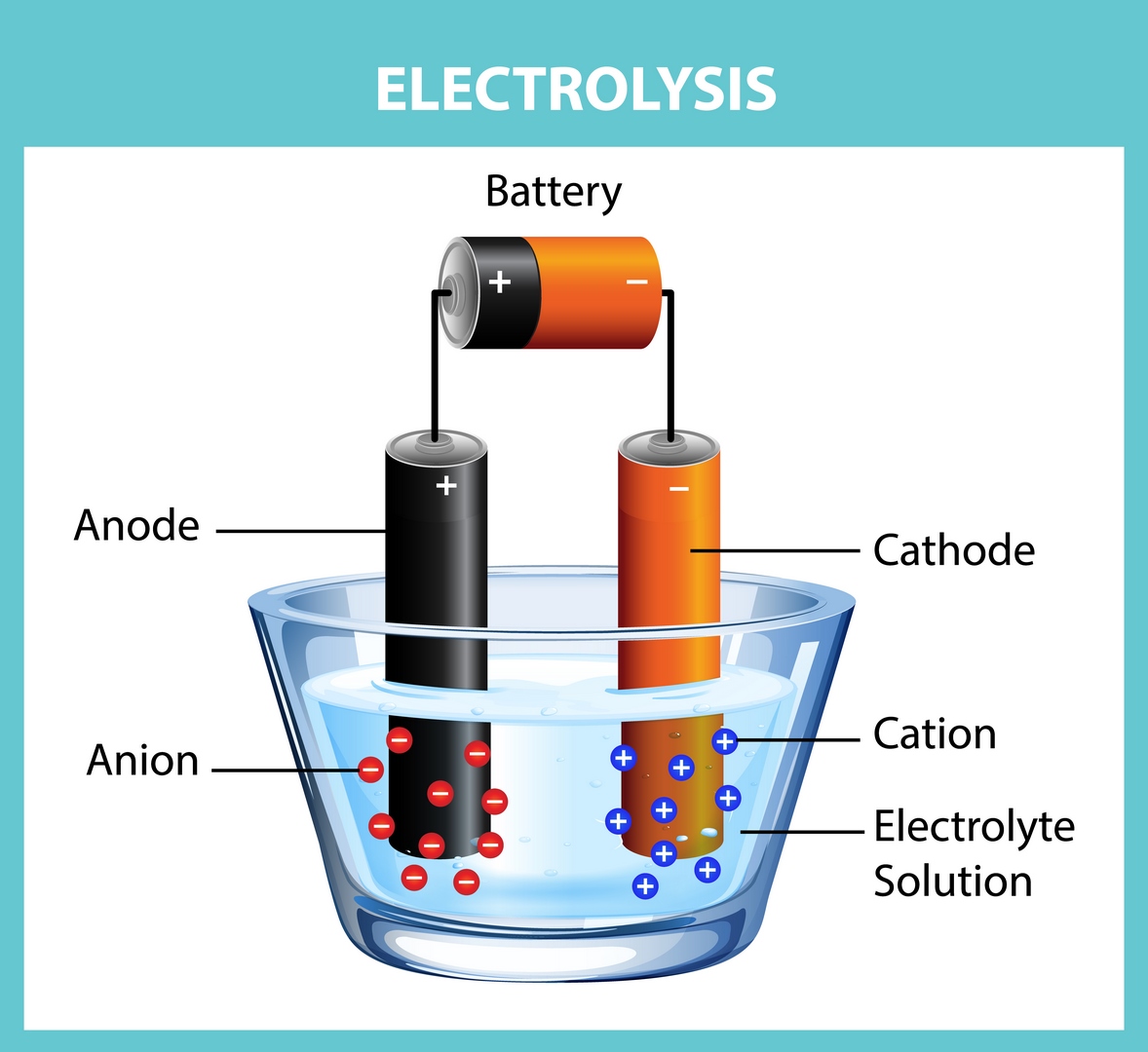
The introduction of electrolytes is a crucial step in the assembly line process for lithium batteries, as it involves incorporating a conductive solution that enables ion transport within the battery for efficient operation.
Electrolytes play a vital role in facilitating the movement of ions between the positive and negative electrodes, allowing for the flow of electrical current. Typically, lithium-ion batteries use liquid electrolytes consisting of lithium salts dissolved in organic solvents. These solvents provide high conductivity and are capable of dissolving lithium salts effectively.
During the introduction of electrolytes, precision, and accuracy are paramount to ensure optimal battery performance. The electrolyte must be carefully injected into each cell or pouch to achieve uniform distribution throughout the battery.
This process requires sophisticated equipment and control systems to maintain consistent volume and prevent any leaks or air bubbles that could compromise the integrity of the battery. Additionally, manufacturers must adhere to strict safety measures when handling electrolytes, as they can be flammable and hazardous if mishandled.
(4) Sealing and Encapsulation
Sealing and encapsulation are critical steps in the assembly line process for lithium batteries, ensuring the protection and integrity of the battery components. This process involves enclosing the battery cells within a protective barrier to prevent external contaminants from entering and causing damage or degradation to the internal components. It also helps to maintain the stability of the electrolyte solution inside the battery.
During sealing and encapsulation, several important steps are taken to ensure a secure and reliable seal:
- Welding: The first step involves welding or fusing together various components of the battery enclosure, such as metal cans or caps. This creates a hermetic seal that prevents any leakage of electrolytes or gases from inside the cell.
- Insulation: After welding, insulation materials are applied around the welded areas to provide additional protection against physical damage, vibration, and electrical short circuits. These insulation materials may include polymer films or pads that help isolate different parts of the battery.
- Coating: Finally, a protective coating is applied over the entire enclosure to further enhance its resistance against moisture, corrosion, and other environmental factors. This coating can be made of various materials like epoxy resins or specialized polymers that provide an impermeable barrier.
By following this meticulous sealing and encapsulation process, the top lithium battery manufacturers ensure that their products meet stringent quality standards while providing optimal performance throughout their lifespan. This attention to detail ensures that users have access to high-quality batteries that offer reliability while meeting safety requirements in diverse applications ranging from consumer electronics to electric vehicles.
(5) Formation Cycling
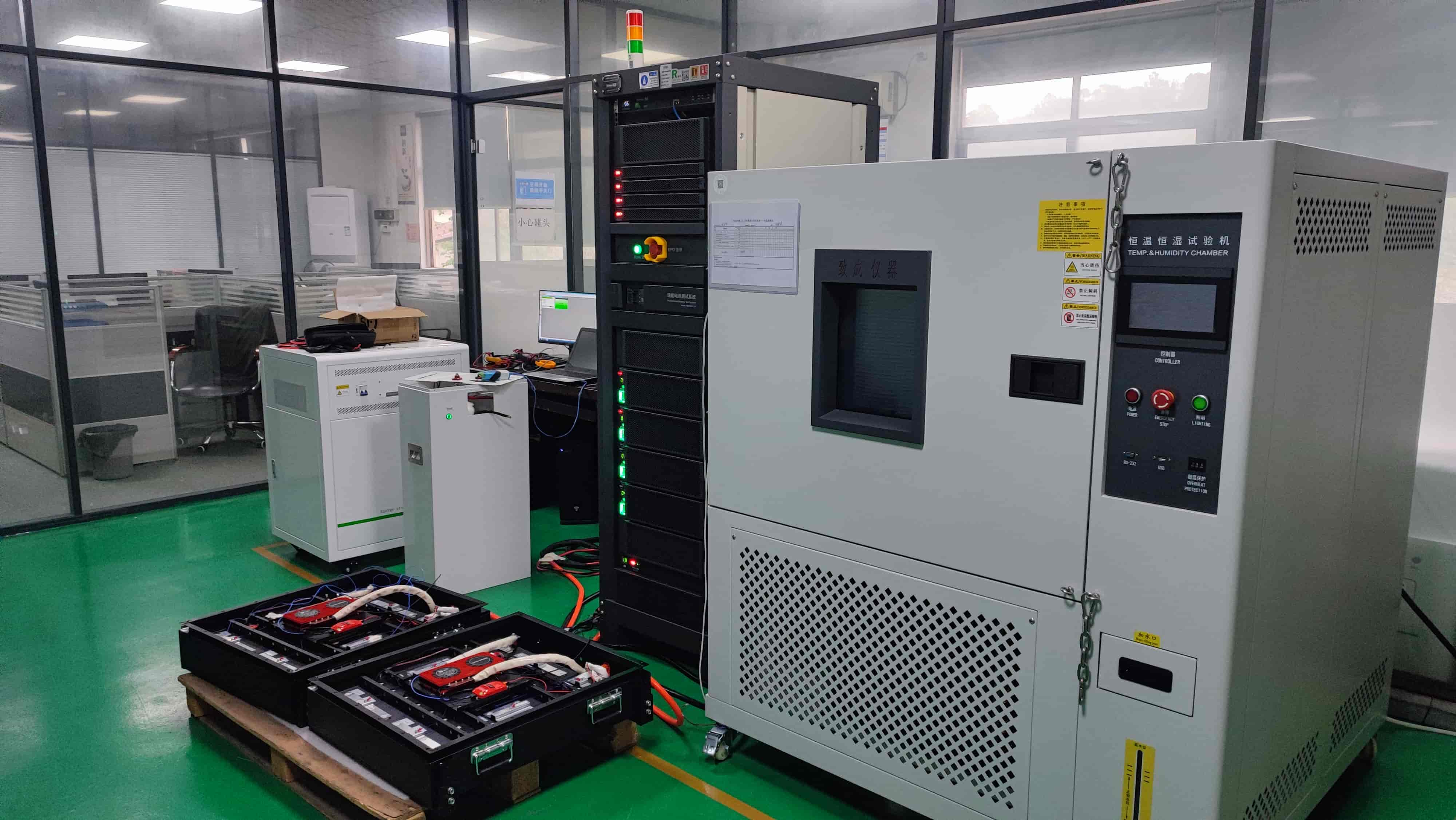
Formation cycling is a crucial step in the assembly line process for lithium batteries, ensuring optimal performance and capacity by subjecting the cells to a series of charge and discharge cycles before they are ready for use.
This process involves charging the battery to its maximum capacity, followed by discharging it completely. These charge and discharge cycles help activate the various components within the battery, such as the cathode and anode materials, electrolyte, and separator.
During formation cycling, any impurities or defects present in the battery are identified and addressed. This helps improve the overall efficiency of the battery by eliminating any potential weak spots or areas of reduced performance.
Additionally, formation cycling also aids in stabilizing the battery's voltage output over time, ensuring consistent power delivery throughout its lifespan.
The goal of formation cycling is to optimize both the energy density and cycle life of lithium batteries. By subjecting them to this rigorous testing process early on in their production, manufacturers can identify any potential issues or weaknesses before they reach consumers.
This not only improves performance but also enhances safety by minimizing the risk of malfunctions or failures during usage.
Section 4: Testing and Quality Assurance
(1) Performance Evaluation
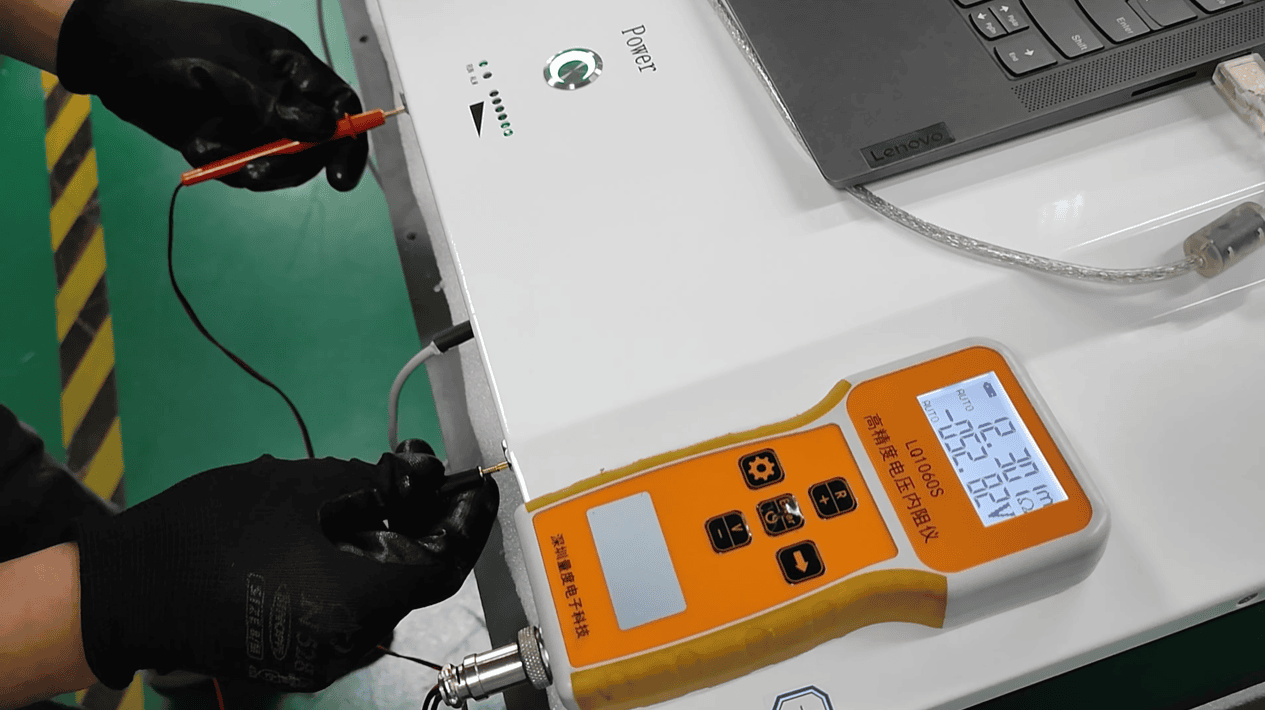
Performance evaluation during lithium battery manufacturing ensures that each unit meets stringent quality standards for capacity, voltage stability, and cycle life.
One key test is the measurement of voltage (OCV), which indicates the potential difference between the positive and negative terminals when no current is flowing. OCV provides crucial information about the state of charge and the health of the battery cell.
Another key aspect evaluated during this test is capacity, which refers to the amount of charge a battery can store. Capacity testing helps manufacturers understand how much energy can be stored and delivered by the battery under different conditions.
The battery internal resistance test is also important. By measuring this resistance, it is possible to assess how efficiently energy can be transferred within the battery cell.
Additionally, cycle life testing is conducted to determine how many charge-discharge cycles a lithium battery can withstand before its capacity significantly degrades.
Lastly, safety tests such as thermal stability analysis are performed to ensure that the battery cells do not pose any risk of overheating or catching fire.
Through these rigorous assessments, the top lithium battery manufacturers strive to produce high-quality batteries that meet both performance requirements and consumer expectations while ensuring safety throughout their lifecycle.
(2) Safety Testing
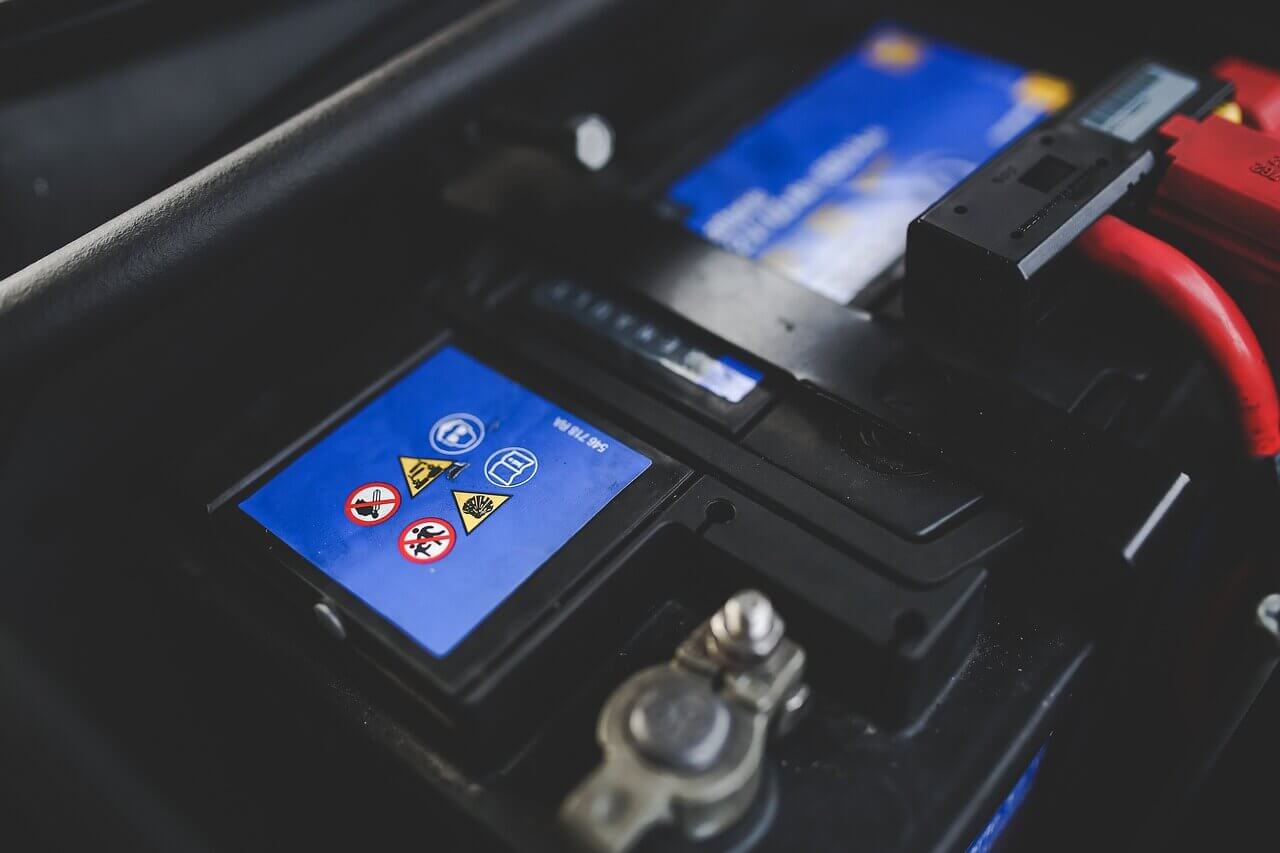
Safety testing is an integral part of the manufacturing workflow for lithium batteries, as it ensures that potential risks associated with temperature, overcharge, and short circuits are identified and mitigated.
Temperature testing involves subjecting the batteries to extreme conditions to evaluate their performance and stability. This includes exposing them to both high and low temperatures to assess their ability to function safely in various environments.
Overcharge testing is conducted to determine the battery's response when subjected to excessive charging currents or prolonged charging periods. By evaluating how the battery behaves under these conditions, manufacturers can identify any potential safety hazards such as thermal runaway or electrolyte decomposition.
Short circuit testing is another crucial aspect of safety evaluation in lithium battery manufacturing. It involves intentionally creating a direct connection between the positive and negative terminals of a battery cell using a conductive material.
This test helps assess the battery's ability to withstand internal or external short circuits without experiencing catastrophic failure or thermal runaway. Manufacturers analyze parameters such as current flow, voltage drop, and temperature changes during this process.
(3) Quality Control Measures
In the previous subtopic, we explored the safety testing procedures conducted during the lithium battery manufacturing process. These tests include evaluating temperature performance, overcharge protection, and short circuit prevention to ensure the safe operation of these batteries.
Now, let us delve into another crucial aspect of this process: quality control measures.
Quality control is a fundamental aspect of lithium battery manufacturing that ensures consistent performance and reliability. By implementing rigorous quality control measures throughout every stage of production, manufacturers can identify and rectify any defects or inconsistencies in their products. Several key quality control measures are employed, including:
- Visual Inspection: This involves carefully examining each battery for any physical defects such as dents, leaks, or improper connections. Manufacturers employ advanced imaging techniques and automated systems to detect even minor imperfections that may affect battery performance.
- Electrochemical Testing: To assess the electrical characteristics of batteries accurately, various electrochemical tests are performed. These tests evaluate parameters like capacity, internal resistance, voltage profiles under load conditions, and cycle life to ensure optimal energy storage capabilities.
- Material Analysis: Quality control also includes analyzing the composition and purity of materials used in battery components such as electrodes and electrolytes. This analysis helps identify any impurities or variations that might impact battery performance or safety.
Section 5: Battery Pack Assembly
This paragraph will discuss the key points related to battery pack assembly in the lithium battery manufacturing process.
(1) Integration of Cells into Packs
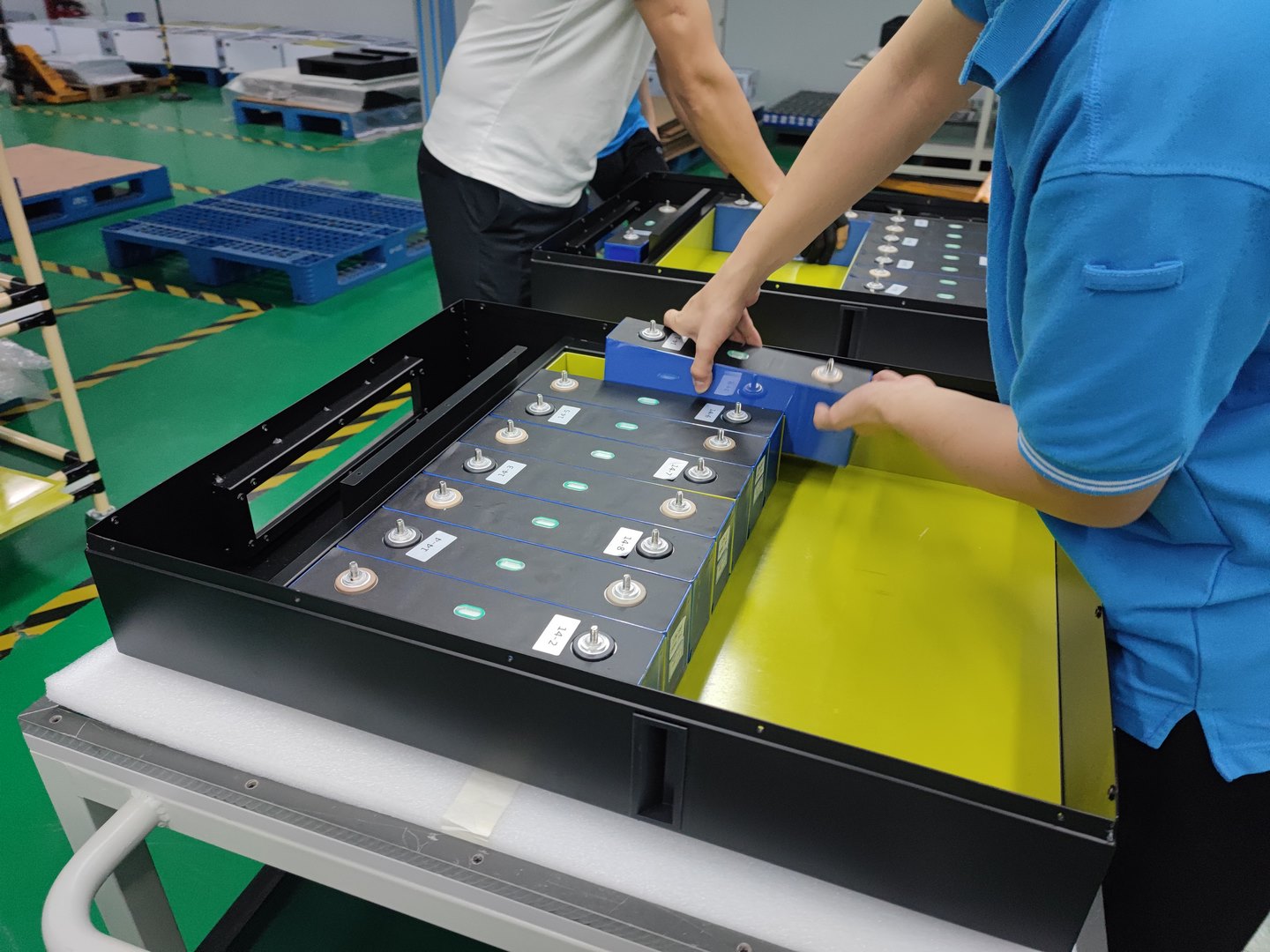
The process of integrating individual battery cells into packs requires careful consideration of factors such as module design, electrical connections, and safety mechanisms.
Module design plays a crucial role in ensuring the efficient and effective integration of battery cells. The modules are designed to accommodate a specific number of cells and provide structural support while also allowing for proper cooling and thermal management. Additionally, the design should consider factors such as weight distribution, space utilization, and ease of assembly.
Electrical connections between the battery cells within a pack are critical for achieving optimal performance. These connections must be carefully designed to minimize resistance and ensure uniform current distribution among the cells. Various techniques such as spot welding or ultrasonic welding can be employed to establish reliable electrical connections between the cell terminals.
(2) Thermal Management Systems
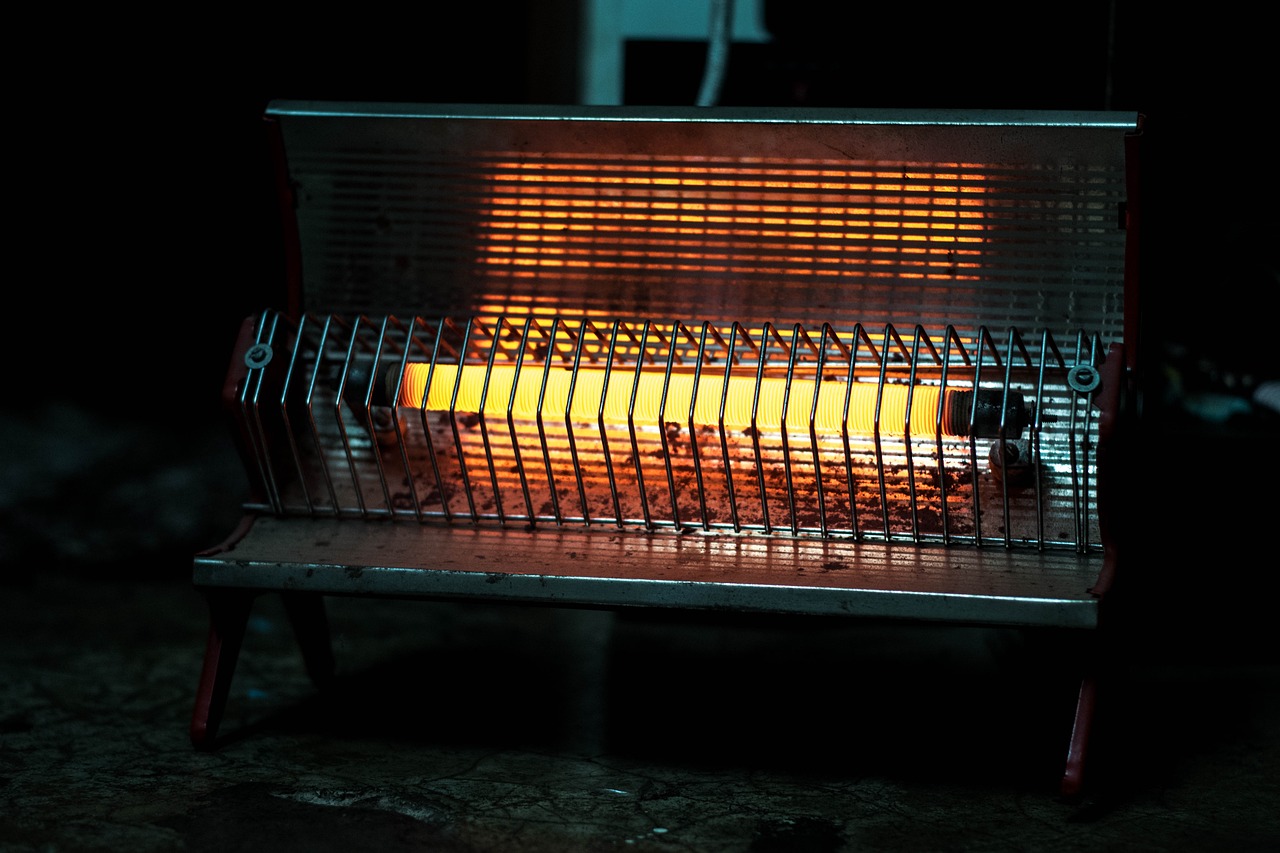
Thermal management systems play a crucial role in ensuring the efficient operation and longevity of integrated battery packs, as they help regulate temperature levels and prevent excessive heating that could lead to performance degradation or safety hazards.
These systems are designed to dissipate heat generated during battery charging and discharging processes, maintaining the cells within optimal temperature ranges.
One common approach is the use of passive cooling methods, such as heat sinks or thermally conductive materials, which transfer heat away from the cells to the surrounding environment. Additionally, active cooling techniques may be employed, such as liquid or air cooling systems, which actively remove heat from the battery pack through circulation.
To achieve effective thermal management, various factors need to be considered. The design of the pack should include proper spacing between cells to allow for efficient airflow or coolant circulation. Furthermore, insulation materials may be applied to minimize heat transfer between adjacent cells or external environments.
Moreover, advanced thermal management systems incorporate sensors and control algorithms that monitor temperature variations in real-time and adjust cooling mechanisms accordingly. These intelligent systems enable precise regulation of temperature levels within narrow limits, optimizing battery performance while ensuring safety.
(3) Battery Management Systems
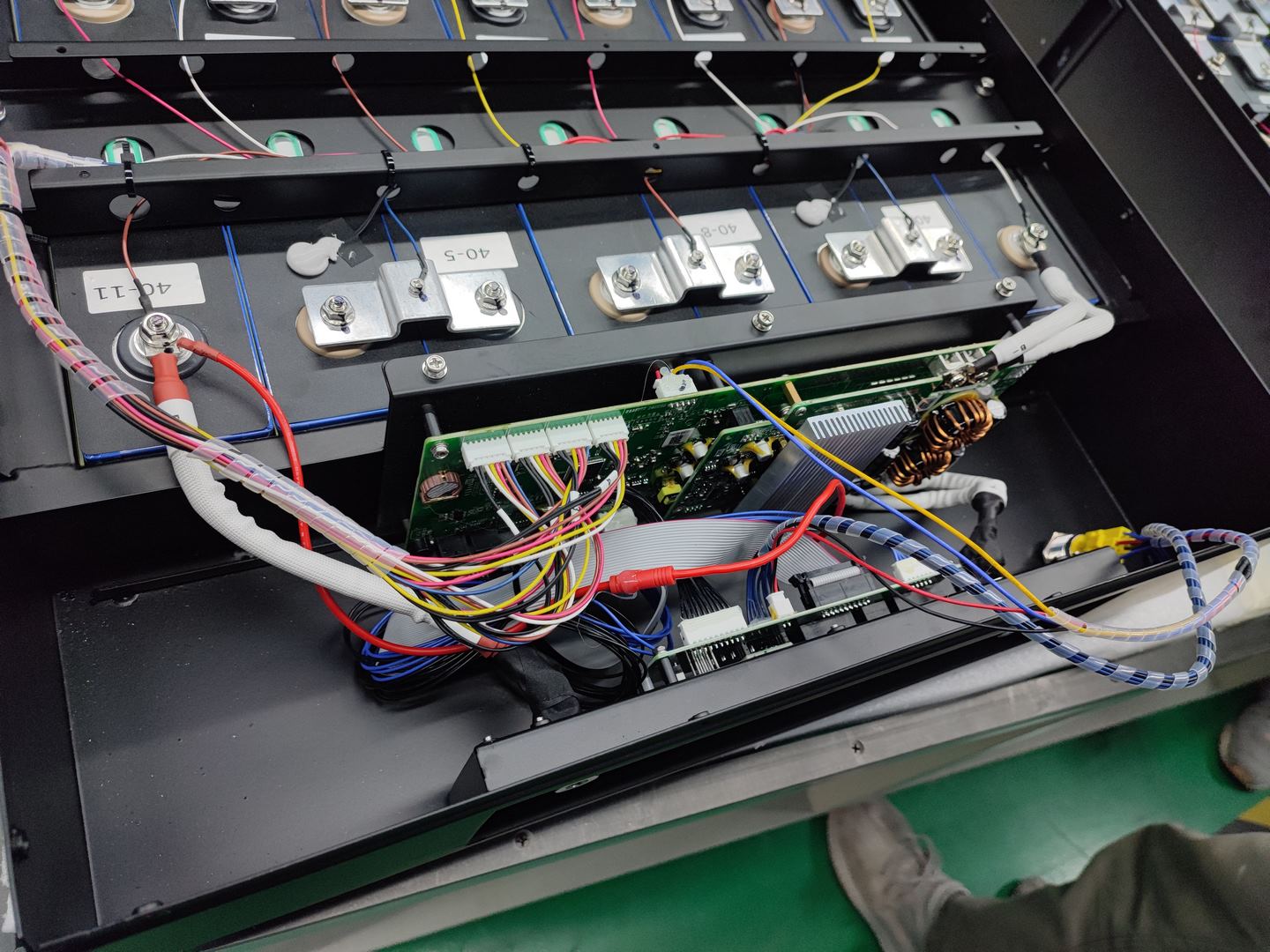
Battery Management Systems (BMS) play a pivotal role in ensuring the efficient and safe operation of integrated battery packs by monitoring crucial parameters and controlling various functions to optimize performance and longevity.
A BMS is responsible for monitoring the state of charge, state of health, and state of function of each individual cell within a battery pack. It constantly measures voltage, current, temperature, and other relevant parameters to ensure that each cell operates within its safe limits.
By continuously monitoring these parameters, the BMS can detect any abnormalities or deviations from optimal functioning and take corrective actions to mitigate potential risks.
In addition to monitoring the cells, a BMS also manages various functions to optimize performance and longevity. It controls charging processes by regulating the current flow into the battery pack, preventing overcharging or undercharging which can lead to degradation or reduced capacity.
The BMS also balances the charge levels among individual cells to prevent some cells from becoming overcharged while others remain undercharged. This balance ensures uniform wear across all cells and maximizes overall capacity utilization.
Furthermore, the BMS provides protection against overcurrents, short circuits, overheating, and other potentially hazardous events that could compromise safety or damage the battery pack.
Top South African Lithium Battery Manufacturers
Power your life with confidence using HARVEYPOW Lifepo4 Battery Pack. Our batteries offer unrivaled advantages that will redefine your energy experience. Here's why:
- Unbeatable Warranty: Enjoy peace of mind with our industry-leading 12-year warranty. We stand behind the quality and longevity of our batteries.
- Superior Quality: Crafted with CATL brand batteries, renowned as the best in the world, our products deliver unmatched performance and durability.
- Endless Power Cycles: Experience the freedom of up to 8000 cycles, ensuring your battery lasts longer and delivers consistent power for years to come.
- Seamless Compatibility: Our Lifepo4 battery pack effortlessly integrates with over 30 inverter brands, offering versatility and compatibility for your specific needs.
- Cutting-Edge Technology: Powered by our intelligent automatic production line and precision laser welding technology, our batteries are built with utmost precision and reliability.
- Customer Support: 7 days to 24 hours, providing professional lithium battery maintenance and usage skills
Choose HARVEYPOW for batteries that redefine excellence. Don't settle for less when you can power up your life with the best. Embrace the future of energy with HARVEYPOW Lifepo4 Battery Pack today!
Section 6: Advancements in Battery Manufacturing Technologies
This discussion will focus on advancements in battery manufacturing technologies, specifically solid-state batteries, lithium-sulfur batteries, new electrode coating methods, and advanced manufacturing equipment.
Solid-state batteries are being developed as a promising alternative to traditional lithium-ion batteries due to their higher energy density and improved safety.
Lithium-sulfur batteries have also gained attention for their potential to deliver higher energy densities and lower costs.
Additionally, new electrode coating methods and advanced manufacturing equipment are being explored to enhance the efficiency and scalability of battery production processes.
Solid-State Batteries
Solid-state batteries have emerged as a promising alternative to traditional lithium-ion batteries due to their potential for higher energy density and improved safety. These batteries utilize solid electrolytes instead of liquid or gel electrolytes found in conventional lithium-ion batteries.
Solid-state batteries offer several advantages that make them an attractive option for various applications:
- Enhanced energy density: Solid-state batteries can store more energy per unit volume compared to traditional lithium-ion batteries. This increased energy density allows for longer-lasting power sources, making them ideal for electric vehicles and portable electronic devices.
- Improved safety: The use of solid electrolytes eliminates the risk of leakage or thermal runaway associated with liquid or gel electrolytes in lithium-ion batteries. Solid-state batteries are less prone to catching fire or exploding, enhancing overall safety.
- Faster charging times: Solid-state battery technology enables faster charging times compared to conventional lithium-ion batteries. This feature is particularly beneficial for electric vehicles, as it reduces downtime during recharging and improves overall convenience.
- Longer lifespan: The inherent stability of solid-state battery materials contributes to a longer lifespan compared to traditional lithium-ion batteries. This longevity makes solid-state batteries a cost-effective option, as they require fewer replacements over time.
- Flexibility in design: Unlike liquid or gel electrolyte-based systems, solid-state battery technology offers flexibility in design and form factor. Manufacturers have the freedom to create custom shapes and sizes, allowing for better integration into various devices and applications.
Lithium-Sulfur Batteries
Lithium-sulfur (Li-S) batteries are another promising alternative to conventional lithium-ion batteries. They operate based on a reversible redox reaction between lithium and sulfur, where sulfur acts as the cathode material. Li-S batteries offer several advantages over their counterparts, including high theoretical energy density (approximately 2600 Wh/kg), low cost due to abundant sulfur resources, and environmental friendliness.
To further illustrate the benefits of Li-S batteries compared to traditional lithium-ion ones, we can look at a comparison table:
Characteristic | Lithium-Ion Batteries | Lithium-Sulfur Batteries |
---|---|---|
Energy Density | Moderate | High |
Cost | Relatively high | Low |
Environmental Impact | Concerns about rare earth elements used | Abundant sulfur resources |
Safety | Risk of thermal runaway | Improved safety due to lower reactivity |
New Electrode Coating Methods
and exploration in the realm of lithium battery technology, offering potential advancements in energy storage systems. These methods involve applying various coatings to the electrodes of lithium batteries to enhance their performance and lifespan.
Some notable developments in this field include:
- Thin Film Coating: One technique involves depositing a thin film coating on the electrode surface to improve its stability and prevent side reactions with the electrolyte. This can help mitigate issues such as capacity fade and dendrite formation, which are common problems in lithium batteries.
- Protective Coatings: Another approach is to apply protective coatings that act as barriers against moisture, oxygen, and other contaminants. These coatings not only increase the battery's resistance to degradation but also enhance its safety by reducing the risk of thermal runaways or short circuits.
- Conductive Coatings: To improve electrical conductivity within the battery, researchers have been exploring different types of conductive coatings for electrodes. These coatings facilitate efficient electron transfer between the active materials and current collectors, thus enhancing overall battery performance.
- Self-Healing Coatings: A more recent development is self-healing coatings that can repair small cracks or damage on the electrode surface autonomously. These coatings contain microcapsules filled with healing agents that are released upon damage, effectively restoring the structural integrity of the electrode and prolonging battery life.
Advanced Manufacturing Equipment
One notable aspect of the development of advanced manufacturing equipment is its potential to revolutionize the production and assembly of components in energy storage devices. These cutting-edge machines and technologies are designed to enhance efficiency, accuracy, and speed in the manufacturing process, ultimately leading to improved battery performance and reduced costs.
For instance, one such piece of equipment is the automated electrode stacking machine that can precisely stack multiple layers of electrodes with minimal human intervention. This not only eliminates human errors but also increases productivity by reducing assembly time.
Additionally, advanced robotic systems equipped with artificial intelligence algorithms can be employed for quality control checks during the manufacturing process, ensuring consistent output and minimizing defects.
Furthermore, the use of laser welding technology enables precise and reliable joining of battery components while improving overall battery performance.
Overall, these advancements in manufacturing equipment hold great promise for achieving higher levels of automation and precision in lithium battery production, paving the way for more efficient energy storage solutions that align with our subconscious desire for freedom.
Frequently Asked Questions
How long does it take to manufacture a lithium battery from start to finish?
The manufacturing process of a lithium battery typically takes several days to weeks, depending on various factors such as the type and size of the battery. This intricate process involves assembling components, electrolyte filling, sealing, testing, and quality control measures.
What are the 3 main components of a lithium battery?
The main components used in the production of lithium batteries include a cathode, an anode, and an electrolyte. These components work together to store and release energy, making lithium batteries essential for various applications.
Are there any environmental concerns associated with the manufacturing process of lithium batteries?
Yes, there are environmental concerns associated with the manufacturing process of lithium batteries. These include resource extraction, energy-intensive production processes, and the disposal of used batteries, which can release harmful chemicals into the environment if not properly managed.
How do lithium battery manufacturers ensure the safety and reliability of their products?
The top lithium battery manufacturers in china ensure safety and reliability through rigorous quality control measures. They conduct extensive testing, such as thermal cycling and abuse tests, to identify potential issues. Additionally, strict manufacturing standards are followed to minimize the risk of defects and ensure product performance.
What is the disadvantage of lithium batteries?
Limited Energy Density: Compared to other battery chemistries, lithium batteries have a relatively lower energy density, which affects their capacity to store energy.
Safety Concerns: Lithium batteries can be prone to thermal runaways and fires if not handled or manufactured properly.
Environmental Impact: Although efforts are being made to reduce the environmental impact, the extraction and disposal of raw materials, as well as the production processes, can have negative consequences.
What is the difference between a lithium battery and a lithium-ion battery?
The terms "lithium battery" and "lithium-ion battery" are often used interchangeably, but technically, there is a distinction:
Lithium Battery: This term typically refers to disposable lithium batteries, also known as primary lithium batteries. They are non-rechargeable and commonly found in devices like cameras and watches.
Lithium-ion Battery: This term refers to rechargeable lithium batteries. They are commonly used in various devices, including smartphones, laptops, and electric vehicles. Lithium-ion batteries offer the advantage of being rechargeable and have a higher energy density compared to disposable lithium batteries.
Learn More Top Questions About Lithium Batteries!
Conclusion
In conclusion, the lithium battery manufacturing process is a complex and intricate endeavor that requires careful attention to detail and adherence to strict quality control measures. From the production of individual cells to the assembly of battery packs, every step in the process plays a crucial role in ensuring the final product's performance and reliability.
Throughout this article, we have explored the various stages involved in lithium battery manufacturing, including cell production and pack assembly. We have also highlighted some of the top South African manufacturers in this industry and discussed the challenges they face as well as their innovative solutions.
Metaphorically speaking, the lithium battery manufacturing process can be likened to constructing a well-built house. Each component must be carefully crafted and assembled with precision to ensure structural integrity and longevity. Just as a sturdy foundation is essential for a house's stability, so too is rigorous quality control necessary for lithium batteries' overall performance.
Innovation continues to drive advancements in battery technology, enabling longer-lasting and more efficient power storage solutions. As demand for these batteries grows across various industries, it becomes increasingly important for manufacturers to find ways to overcome challenges like cost reduction while still maintaining high-quality standards.
Overall, understanding how lithium batteries are manufactured provides valuable insight into their capabilities and potential applications. With ongoing research and development efforts focused on improving efficiency and sustainability, we can expect even greater advancements in this field in the future.